Until Now
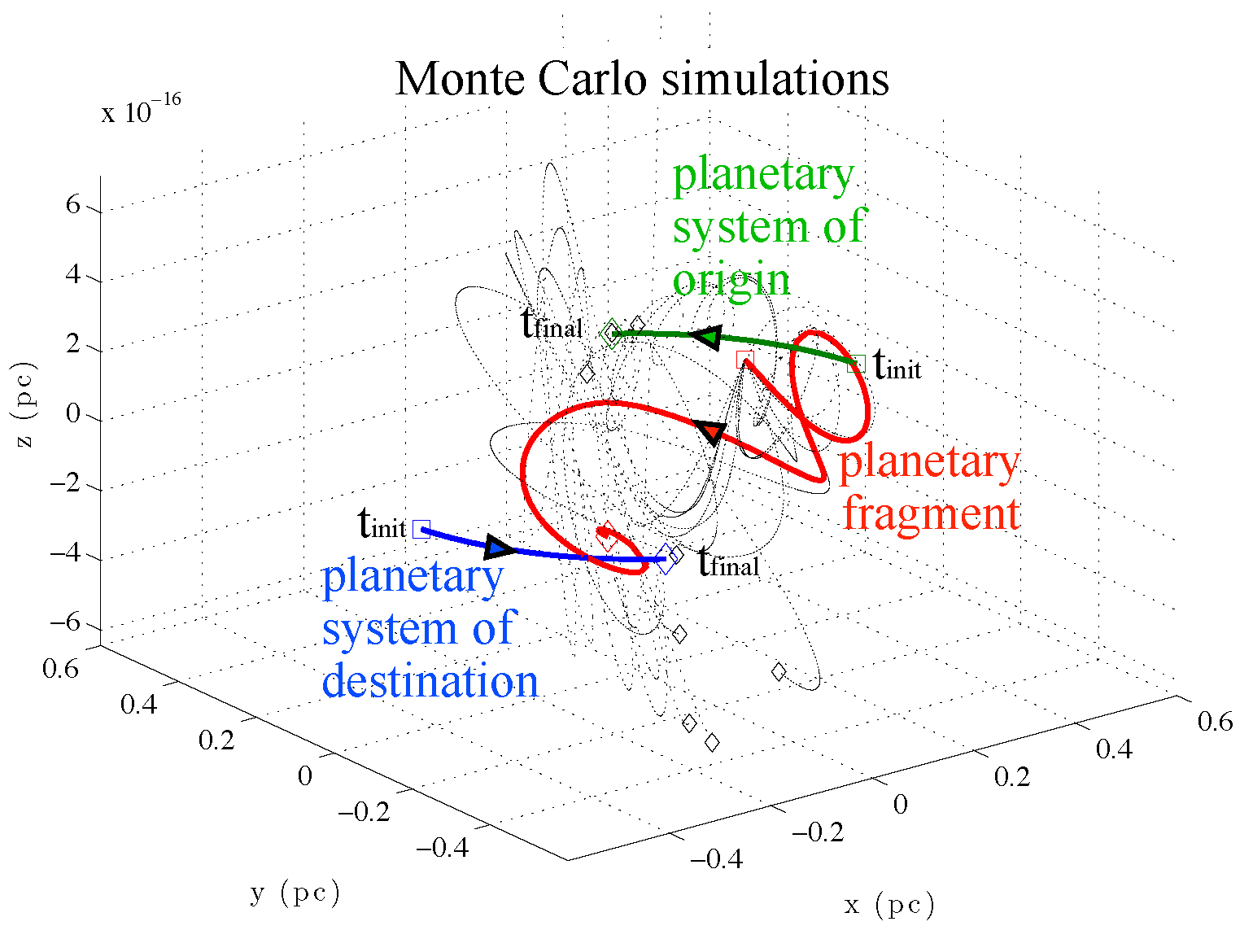
Encouraged by the high frequency of planetesimals around solar-type stars, we revisited the issue of the transfer of debris between young nearby planetary systems still embedded in their birth stellar cluster. We found that the transfer probabilities are greatly increased when considering quasi-parabolic, chaotic orbits. The figure to the right (from Belbruno et al. 2012) shows a few examples of the million Monte Carlo realizations carried out, showing the chaotic trajectory of a meteoroid that is successfully transferred between two stellar neighbors (in red), and others that are not transferred (dotted black lines).
Timescales are critical
For lithopanspermia to be a viable hypothesis, we not only need an efficient transfer mechanism (discussed above), but we also need life to have developed on Earth before the cluster dispersed (after which the transfer probabilities would become too low). Is there evidence that this could have been the case? From the isotopic ratio of oxygen found in zircons, there are indications that liquid water was present in the crust of the Earth
when the solar system was as young as 164 or 288 million years old, depending on the study, which indicates that
habitable conditions might have been present early on. Other studies show that the isotopic ratio of
carbon in old sedimentary rocks indicates evidence of biological activity when the solar system was
only 718 million years old. The timescales for abiogenesis (the natural process of life arising from non-living matter) are thought to range from 0.1–1 million years for hydrothermal conditions at the deep sea, to 0.3–3 million years for warm-puddle conditions in shallow water, to 1–10 million years for subaeric
conditions in the soil—all at least an order of magnitude less than the lifetime of the stellar cluster.
If life arose on Earth shortly after liquid water was available on its crust, the window of opportunity
for life-bearing rocks to be transferred to another planetary system in the cluster opens when liquid
water was available (when the Solar system was 164–288 million years old), and ends by the cluster dispersal time (when the Solar system was 135–535 million years old; see bottom and top timelines in the figure below).
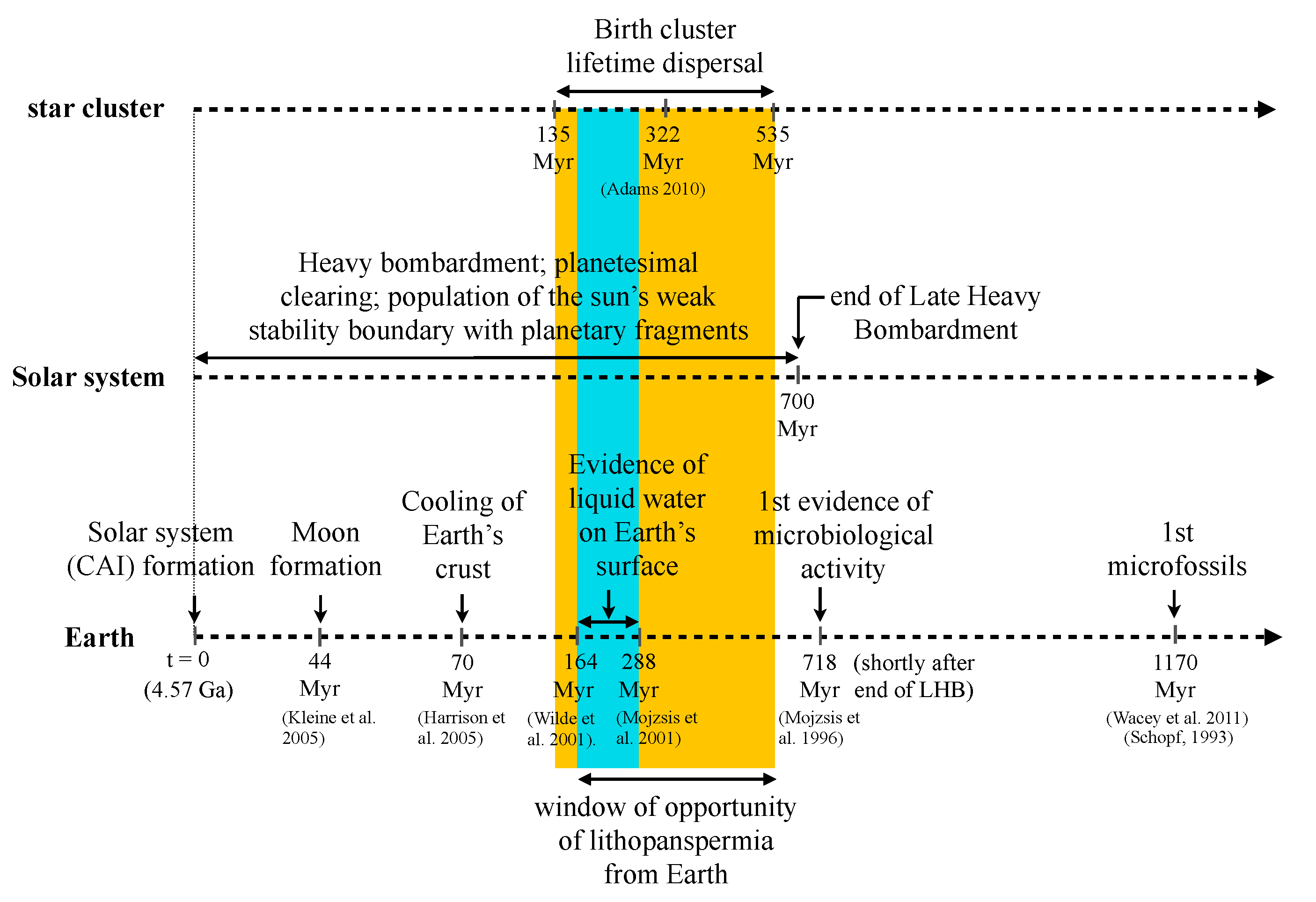
Not only there is this window of opportunity, but there is evidence that heavy bombardment ejected large quantities of possibly life-bearing rocks from Earth, ejecting them to outer space. This
bombardment period lasted from the end of the planet–accretion phase until the end of the late heavy
bombardment 3800 million years ago, when the solar system was approximately 770 million old.
The bombardment is also evidence that planetesimals were being cleared from the solar system several
hundred million years after planet formation. This period of massive bombardment and planetesimal ejection from the Solar system completely encompassed the
“window of opportunity” for the transfer of life-bearing rocks, providing a viable ejection mechanism for lithopanspermia (see middle timeline in the figure above).
But there is one final consideration to assess if lithopanspermia could be viable: Is the time for survival of microorganisms in deep space longer than the characteristic transfer timescale? In other words, could microorganisms surive long interstellar journey? Microorganisms can be sheltered from the hazards
of outer space (ultraviolet light, x-rays and cosmic rays) if hidden below the surface of the rocks.
Survival for millions of years cannot be tested with direct experiments (e.g., experiments testing the
survival on the surface of the Moon lasted only a few years), but there are computer simulations that
model the conditions in outer space for extended periods of time. Based on these studies and comparing to
the timescales involved in the weak-transfer mechanisms, including the time it might take to land on
a terrestrial planet, we found that microorganisms could survive the long interstellar journey hidden in
meteoroids larger than about one meter.
All the considerations above indicate that it is therefore possible that life on Earth could have been transferred to other planetary systems when
the Sun was still embedded in its stellar birth cluster. But could life on Earth have originated beyond the
boundaries of our solar system? Our results indicate that, from the point of view of dynamical transport
efficiency, life-bearing extrasolar planetesimals could have been delivered to the solar system if life had a sufficiently early start in other planetary systems, before the
solar maternal cluster dispersed. An early microbial biosphere, if it existed, would likely have survived
the Late Heavy Bombardment. Thus, both possibilities remain open: that life was “seeded” on Earth
by extrasolar planetesimals, or that terrestrial life was transported to other star systems via dynamical
transport of meteorites. Regarding the search for life beyond our solar system, this opens a new world
of possibilities to dream about, given how many extra-solar planetary systems are out there and how
tremendously diverse they are.
Adapted from an article that appeared in the Space Telescope Science Institute Newsletter (Winter 2015), based on results published in Belbruno, E., Moro-Martín, A., Malhotra, R., & Savransky, D. 2012, Astrobiology 12, 754